Today’s advances in electron microscopy would not have been possible without a major breakthrough which occurred in the late 1990s and early 2000s: aberration correction.
To explain the significance of this revolution, Oxford professor Peter Nellist, President of the Royal Microscopical Society, talks through the history of the microscope: ‘Microscopy as a scientific technique was really put onto the main scientific agenda in the 17th century, when Robert Hooke published the Micrographia.’ This volume reproduced detailed drawings of the images that Hooke saw through the newly invented microscope, including ants and fleas.
Other developments followed – Von Leeuwenhoek, the founder of microbiology, was able to observe parasites and bacteria. As Professor Nellist puts it, ‘People thought: great, we can see smaller and smaller things – it’s simply a case of making better and better lenses.’
People thought: great, we can see smaller and smaller things – it’s simply a case of making better and better lenses.Professor Peter Nellist
It was only in the late 19th century that Ernst Abbe, working for Zeiss, realised this was not true. Professor Nellist explains: ‘the smallest thing you can see through a microscope is dictated by the wavelength of the radiation you’re using to look at it.’ Light and electrons both act as waves.
‘With light, the typical wavelength is about half a micron. That means you can see cells, and a lot of biological structures, but if you want to look at atoms, you have no chance.’
It was in 1930s Germany that Ernst Ruska realised that a magnetic field could be used as a lens for electrons. The electron microscope, which could look beyond the wavelength of light, was born.
Next, however, came what Professor Nellist calls ‘a horrible irony: that the form of magnetic fields has to obey the laws of physics. And you cannot form a magnetic field into a perfect lens shape, because the laws of physics forbid it.’ This effectively meant that the potential of the very short wavelengths afforded by electrons could not be fully unlocked, because the lenses were not good enough.
The only way to improve the resolution was to build giant, high-voltage instruments, 10 metres tall, weighing 30 tonnes, sitting in 2 rooms.Professor Angus Kirkland
Angus Kirkland, Oxford’s JEOL Professor of Microscopy, draws an analogy between the best magnetic lenses before correction and looking through the curved bottom of a champagne bottle. He recalls: ‘The only way to improve the resolution was to build giant, high-voltage instruments, 10 metres tall, weighing 30 tonnes, sitting in 2 rooms. However, at that energy level, almost all materials suffer severe radiation damage, limiting the experiments that could be carried out.’
What changed this, making all of today’s research possible, was aberration correction – which Professor Nellist describes as ‘spectacles for microscopes’, correcting their deficient vision.
Aberration correctors were initially proposed in the late 1940s, but it took 60 years to develop a working system. Indeed, many felt that aberration correction was simply not possible. The technical challenge was huge, with some extremely complex engineering involved in making the optics.
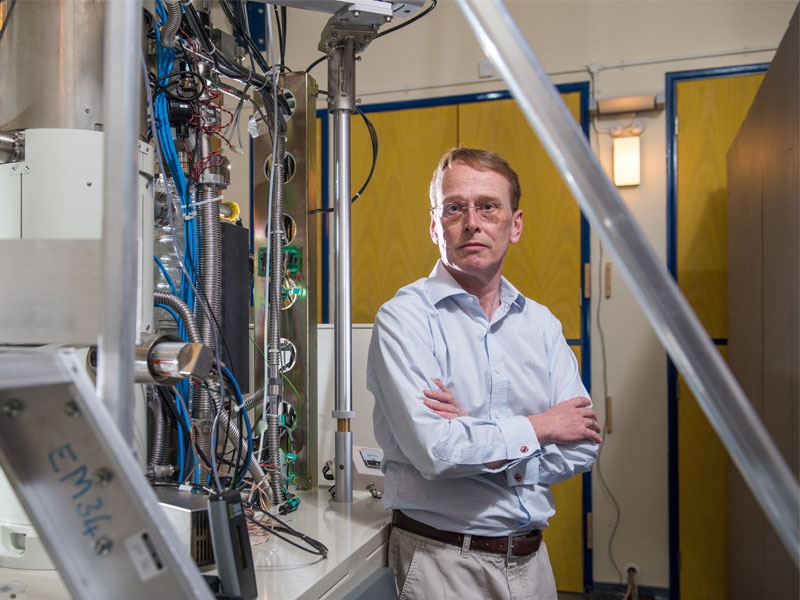
![]()
The ‘corrected’ microscopes themselves were technically involved, requiring between 40 and 50 independent power supplies to run them. Advances in computing power were a contributing factor to achieving an effective solution.
Professor Nellist comments that it was equivalent to ‘having a microscope with 50 knobs, all of which have to be adjusted very carefully to make it work. It’s impossible for a human to do that. One of the keys is that we now have methods and computers that can analyse how our optics are working and take over the control.’
The step change in the capability of the microscope has been so great that we are still understanding ways of making use of this capability.Professor Peter Nellist
Once aberration correctors had been successfully developed, the Oxford group at the time was eager to test the potential of this new technology. Professor Kirkland recalls that with the number of new possibilities it offered, his group had to completely rethink the role of electron microscopy in many of their research areas.
It was Japanese microscope company JEOL which provided them with the first aberration corrected instrument in the UK – and one of the first in the world – as part of its ongoing support of Oxford research.
The future
At Easter 2016, seven lorries arrived near Harwell in rural Oxfordshire, containing two new JEOL aberration-corrected electron microscopes. Located at the Harwell Science and Innovation Campus is Diamond Light Source, the UK’s national synchrotron science facility.
Professor Kirkland, who is also the Science Director for the physical science component, explains about the new Harwell microscopy project: ‘It’s a collaboration centred around one of the synchrotron’s beam lines, which goes out from the storage ring into a separate large building.’
This new facility houses microscopes for life science, funded by the Wellcome Trust and the Medical Research Council, and microscopes for physical science, funded partly by Diamond Light Source themselves, partly by Oxford through JEOL, and partly by Johnson Matthey, a leading UK catalyst company.
Professor Kirkland says, ‘It’s industry, national laboratory and university, all working together. We are going to run it as a user facility, with peer-reviewed applications; users will have access to the instruments and I have a team of expert staff to support them.’
This, he concludes, is the future: ‘it’s the new model – with integrated facilities attached to major national resources such as synchrotrons, and a mixture of life and physical sciences. We take care of the running of these expensive instruments in a national facility, letting the users concentrate on their science.’